Geodesy without math equations: Is that possible? The answer is no, but basic geodetic concepts can be described without using complex math equations.
My previous column addressed the geodesy crisis in the United States. The newsletter was highlighted on LinkedIn (thanks, Jay); more than 235 individuals reacted to the post and there were 25 reposts. (See also xxxx)
I’m pleased so many people are interested in highlighting the discussion of the inverted pyramid. One reader of my column asked for material for non-geodesists to obtain a better understanding of geodetic concepts.
Geodesy does involve advanced mathematics that may not be familiar to some people. That said, there are various online lessons and tutorials that describe the basic concepts without using complex math equations.
As mentioned in my previous column, geodesy is involved with anything related to positioning. For example, have you ever wondered how your phone appears to know where you are on a digital map while you’re walking or driving down the street? Geodesy provides the foundation for all geospatial products and services.

Image: Dave Zilkoski
Location on a Map
A goal of mine has always been to get individuals (young and old) interested in obtaining a better understanding of geodesy. In my opinion, high schools and colleges should include courses that explain to students how their phones know where they are, why the Earth is not a sphere, how the movement of tectonic plates are measured and why, basic concepts of how satellites orbit the earth, and how geographic coordinates are important to making maps and their use in establishing an accurate geographic information system (GIS).
A good first step is to get high school teachers interested in the topic. When I was employed by the National Geodetic Survey (NGS), a group of us worked with local high school students to map their football field using GPS. They acquired observations in the field, and then downloaded the coordinates into their GIS. The teacher was instrumental in integrating the application into the students’ curriculum.
A reader of my last column suggested I provide concrete, meaningful things to lower the barrier of entry. I’m not exactly sure how to lower the barrier of entry — geodesy does require an individual to have a certain level of mathematical knowledge.
Since I retired from NGS, I have helped homeschool my eight grandkids. The one thing that I’ve found is that young students apparently either “like” math or they “hate” math. At least with my grandkids, there doesn’t seem to be an in between.
At this moment, I don’t believe any of my grandkids will become geodesists; well, actually, there’s still a possibility that one may have a “love for mathematics.” It appears that most students don’t really see a reason to learn math. They can use their phones or calculators to do what they need.
The reader suggested that the geodesy community could publish free, high-quality, web-based resources for the public. The reader made the following suggestions:
- A set of 3D-printable designs for rudimentary survey tools; alternatively, how to acquire/build the tools in the most economical way possible. Even something that would be considered a “toy” that can be given to a child would be good.
- A list of software tools (preferably open source) relevant to the subject and how to use them in this context.
- Introductory material intended for young audiences.
This column will provide some free online lessons and tutorials that describe the concepts associated with geodesy and surveying. Some of the online videos are at a level for young audiences, and some are aimed at individuals with more advanced education. Let’s start with the young audience.
Lessons for Kids
The website “Get Kids into Survey” provides materials focused on kids. The website states: “Bringing young people into the exciting world of survey through pioneering content and engaging experiences.” See the boxes titled “Get Kids into Survey Website,” “Get Kids into Survey Website – Poster Page,” and “Get Kids into Survey Website – World Without Surveyors Poster.”
Get Kids into Survey Website
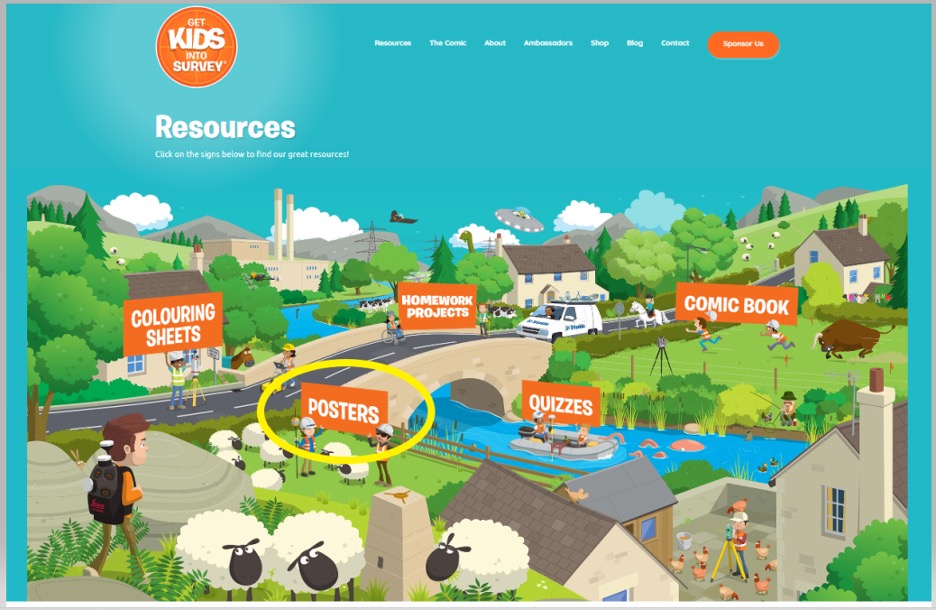
Screenshot: Get Kids lnto Survey
Get Kids into Survey Website – Poster Page

Screenshot: Get Kids into Survey
Get Kids into Survey Website – World Without Surveyors Poster

Screenshot: Get Kids into Survey
The GPS.gov website has lessons describing GPS that are designed for kids. One lesson introduces the concept of GPS trilateration. The lesson explains how GPS positioning works on two basic mathematical concepts:
- trilateration, which literally means positioning from three distances, and
- the relationship between distance traveled, rate (speed) of travel, and amount of time spent traveling.
This was developed by NGS for a National Science Teachers Association Conference. You can download both the instructions and map.’
GPS Trilateration Lesson

Screenshot: GPS.gov website
The following are several videos that describe the concept of trilateration.
This video =explains trilateration and how the GPS ranges (distances from the satellite to the receiver) are computed.
This video uses distances on a map to describe trilateration.
Here is a detailed description of trilateration and why you need the fourth satellite.
Here is a detailed description of how GPS works.
Now, let’s look at some free online lessons and tutorials that describe the concepts associated with geodesy. As previously stated, some of the online videos are at a level for young audiences, and some are aimed at individuals with more advanced education. Most of them describe the concepts using diagrams with narratives, and without complex math equations. NGS provides a number of videos that can be downloaded here.
NGS, in partnership with the COMET program, has developed a series of self-paced lessons on geodetic and remote sensing topics. Users have to create a free user account to gain access to the courses. Users will have the option of printing out a certificate upon successful completion of a quiz at the end of each lesson.
The lessons are rated by skill level ranging from “Suitable for Non-Scientists” to “Requires some Prior Knowledge of the Topic.”
The COMET program provides teaching and training resources for the geoscience community. All of the content is completely free, but an account does need to be created. The COMET program is part of the University Corporation for Atmospheric Research (UCAR) Community Programs.
NGS Online Lessons

Screenshot: NGS Website
NGS and COMET Educational Videos
NGS also has a website that contains educational videos. Again, NGS, in partnership with the COMET Program, has developed short videos on topics related to geodesy and mapping.
NGS Educational Videos

Screenshot: NGS Website
This link provides a tutorial on “Why is geodesy the framework behind all mapping and navigation?” The article states. “If you think about it, the whole field of geomatics lies on the shoulders of geodesists. Because it’s really geodesy that is the framework behind all surveying, mapping and navigation.”
What Is Geodesy?

Screenshot: Gisgeography Website
NASA’s Eratosthenes Estimating the Circumference of the Earth by Looking Down a Well
NASA offers a video titled “Looking Down a Well: A Brief History of Geodesy.” This video explains how it all started when Eratosthenes estimated the circumference of the Earth by looking down a well. It highlights how, over time, the field of geodesy has expanded and evolved dramatically, and how NASA uses technology such as radio telescopes, ground surveys, and satellites to contribute.
NASA’s Video on Looking Down a Well
UNAVCO Measures Plate Tectonics with Geodesy
UNAVCO, a non-profit university-governed consortium, facilitates geoscience research and education using geodesy. UNAVCO has a video that describes the tectonic plates and how geodesists measure their movements. Another UNAVCO video describes what geodesy actually is, as well as geodesy’s application in our everyday lives (UNAVCO’s 2017 USIP geoscience video production). Visit UNAVCO’s website to learn more about its mission.
Geodetic Software Tools
NGS provides tools that focused on meeting the needs of the surveying and mapping community. A few may be of interest to non-geodetic individuals. A map tool can be used to locate marks near someone’s location.
Production NGS Map

Screenshot: NGS Website
UNAVCO also has interactive tools that may be of interest to geospatial users. See the boxes below titled “UNAVCO Interactive Tools” and “UNAVCO Spotlight.”

Screenshot: UNAVCO Website

Screenshot: UNAVCO Website
3D Printer of Surveying Equipment
Now, let’s address the 3D printing of surveying equipment and tools. I’m not familiar with using a 3D printer, but I found several websites that provide information on surveying equipment. Some of the sites provide free information and others charge for their services. See the websites 3D Printer of Total Station and 3D Printer of GNSS Equipment.
3D Printer of Total Station

Screenshot: CULTS Website
3D Printer of GNSS Equipment

Screenshot: 3dmdb Website
I’m pleased a lot of people are interested in highlighting the discussion of the inverted pyramid. As commented by several individuals in the LinkedIn responses, the surveying and photogrammetry (which includes remote sensing) communities are experiencing the same crisis as geodesy. In my opinion, they are all related, because the surveying and mapping community provides tools other disciplines use.
As stated in my last column, the surveying and mapping community can do the following to help:
- Actively market geodesy in high schools as a rewarding career for the math stars before college entry.
- Build back, support and sponsor geodesy programs at select universities. This support needs to be strategic with backing from the highest levels of the U.S. government.
- Encourage U.S. government support in the form of grants, professional development of staff, and research collaborations/affiliations.
As previously mentioned, a goal of mine has always been to get individuals (young and old) interested in obtaining a better understanding of geodesy. I hope this column helps to whet the appetite of some individuals to obtain a better knowledge of geodesy. Maybe even some high school and college teachers will introduce geodetic concepts in their lectures.
Writing about the geodesy crisis is a good first step, but we need to find champions that can influence high school and university teachers and administrators, federal and state government program managers, and congressional representatives.
Please feel free to email me at geospatialsolutionsbyDBZ@gmail.com if you have suggestions on how to lower the barrier of entry into the world of geodesy.